The Science Behind the Sci-Fi Staple: Lasers
- Apr 6, 2018
- 13 min read
Updated: Oct 23, 2023
Written by Harry J. (GAFL'19)
Edited by Ava S. (TBS'19)
━━ April 5, 2018 ━━
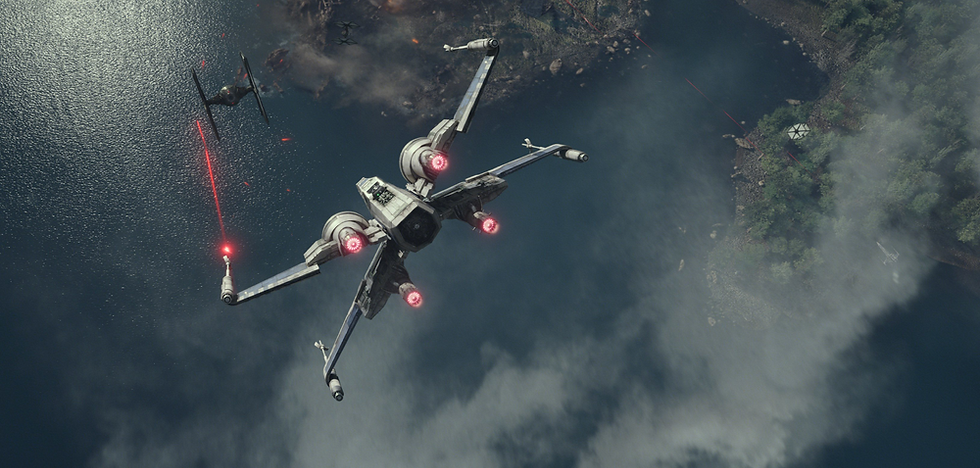
Be it Star Wars or Star Trek, we’ve all seen one of those sci-fi movies; a dazzling affair, where nimble, sleek spaceships shoot each other out of the sky (space, really) with laser guns and massive cannons spewing colorful streaks of light. Despite our geeky wishes otherwise, unfortunately, these will never come to fruition quite in the way as shown on screen, as they are quite physically impossible. However, while devices in such form may exist only in the realm of computer-generated fiction, we may find solace in the fact that they do exist in real life--just not as presented. In this article, we will explore the actual principles behind such devices (and thus see why movie weapons can’t possibly look the way they do on screen) and delve into the real science behind the timeless science fiction staple, the laser.
━━━━━━━━
The Science Behind the Sci-Fi Staple: Lasers
Light
An understanding of light is essential to grasp the concept of lasers. Light is the layman’s term for electromagnetic radiation, which encompasses all wavelengths of electromagnetic waves. It is important to understand that there’s more to light than meets the eye; what we can see is only a tiny part of the full electromagnetic spectrum. Microwaves--the very stuff that heats your food--are a form of light, so is the radio you might listen to on your way to school or work. Wi-fi signals are light. This is the case for a lot of other things--they are all simply different wavelengths of electromagnetic radiation, or light. Shorter wavelengths (higher frequencies) carry more energy than longer wavelengths. (Note: all frequencies of light travel at the same speed.)The brief table below outlines the nomenclature for various frequencies of light.
*Note: λ = c/f (wavelength is the inverse of frequency, where c = speed of light)
1 nm = 10^-9 m
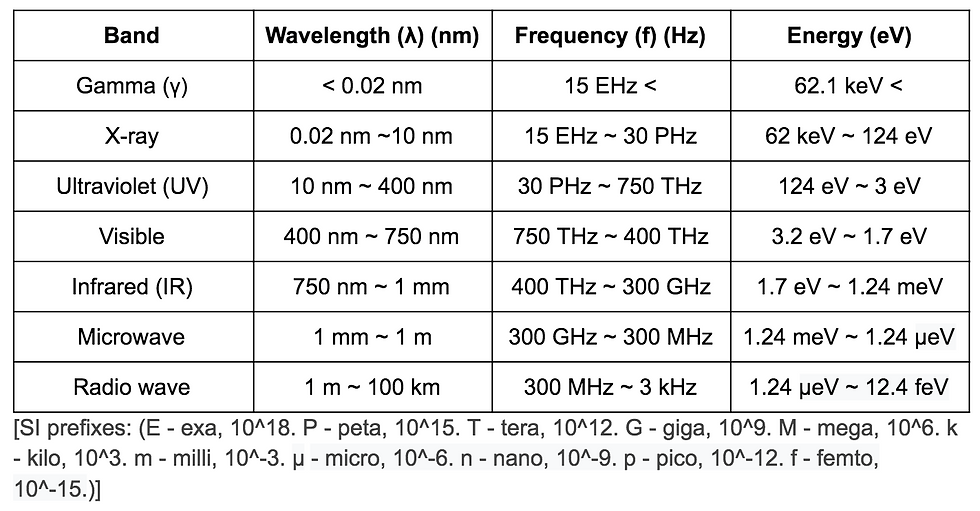
More precise classifications exist, of course, but for everyday use the above serves as the most universal. One can see that wavelength decreases as the frequency increases, as does the energy. The visible spectrum is only a tiny part of this spectrum, with red having the lowest frequency in the visible range, and blue/violet having the highest (and being the most energetic). Prolonged exposure to X-rays is considered dangerous for this reason--each ray carries a small wavelength and a high energy, which is capable of affecting the body at a cellular level. Gamma rays, also known as gamma radiation, are one of the three forms of radiation (alpha radiation being helium nuclei, and beta radiation being electrons), which with its very high frequency and energy is potentially the most hazardous to living tissue. It causes us to exercise extreme caution around radiation and attribute danger to radioactive nuclear fallout. On the other end of the spectrum, we have infrared, whose energy is best converted to heat; this is why heat-imaging sensors sense in the IR spectrum - when something radiates heat, it is actually radiating infrared waves.* This is followed by microwaves, which are used in (you guessed it) microwave ovens. Radio waves cap the other side of the spectrum with a frequency of 3 kHz, and are used for (you guessed it again) radio communications and radar (itself actually an acronym for RAdio Detection And Ranging). Lower frequencies exist, of course, but for all practical purposes, the spectrum of light is considered to range from 3 kHz and above. Lasers serve to take one of these frequencies and shoot it in a straight line.
LASERs
Before you begin blaming me for taking the fun out of everything, I assure you, real lasers are just as fun as ones in the movies. Let’s first dissect how a laser actually works. Lasers are pretty common in everyday life; from the classroom laser pointer to barcode scanners to the DVD reader in your disk drive. The internet cables that snake across the oceans carry laser light laden with information through fiber-optic lines, and at the same time are used to provide visual effects in concerts. More esoteric applications exist, of course--they are also used to kick-start nuclear fusion reactions, as well as find the exact range of the moon or a satellite for calibration purposes. Whatever it may be used for, it is important to understand that all lasers at all frequencies are made for one purpose only--emit a specific frequency of light down a concentrated vector.
The term ‘laser’ is actually an acronym of ‘Light Amplification by Stimulated Emission of Radiation’. You might ask what makes it different from a narrow flashlight. A laser emits light of a very specific frequency based on what it’s made of--more on this in a bit. A laser is distinct from other sources in that it emits light in a spatially and temporally coherent manner; spatially in that it can be concentrated to a tight spot, and temporally in that it emits at a specific wavelength.
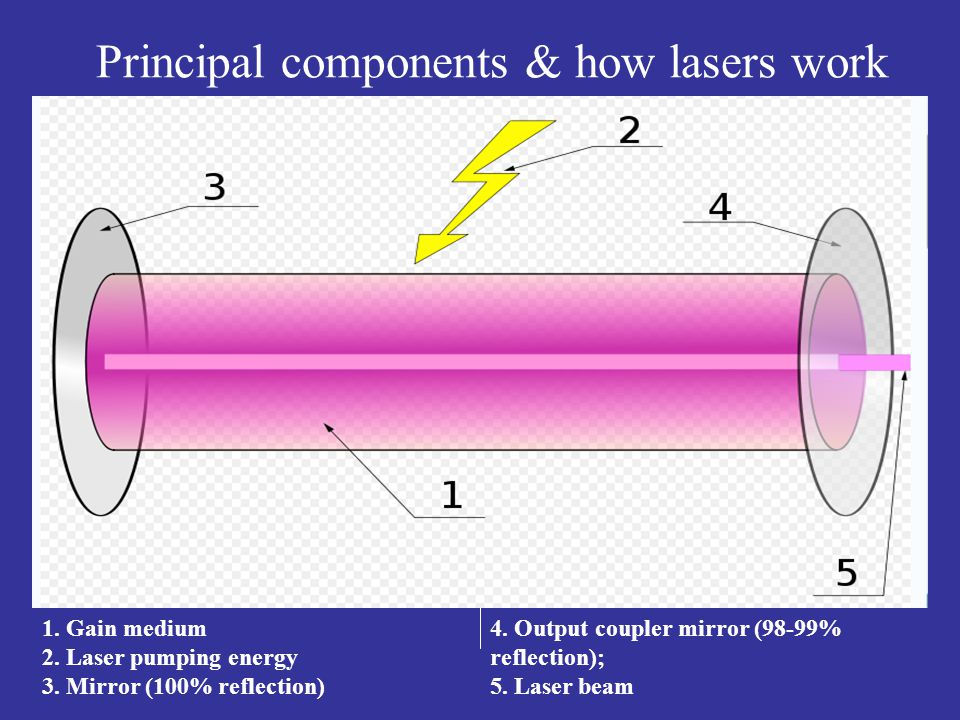
Lasers are composed of three distinct parts: the gain medium (1: material determines frequency), the energy pump (2: source of energy), and something that provides optical feedback (3, 4: bounces the light around in the medium so that it is amplified multiple times), typically a pair of mirrors. The process of supplying energy to the gain medium is known as pumping. This is done either through the form of electrical current, or a light of different frequency from a flash lamp (similar to what is used in camera flashes). One of the two mirrors will be partially transparent, allowing the amplified light to escape in a narrow beam, creating the laser beam as we know it.
Stimulated Emission
The next question one might ask is, ‘how is the resulting beam concentrated on so precise a frequency?’ The reasons behind that lie in the principle of Stimulated Emission, or the S and E of the acronym ‘LASER’. This refers to the interaction between a photon of a specific frequency and an electron of an atom in an excited state. For those who are unfamiliar, I will first explain the principles behind photon emissions from atoms.
Atoms’ electrons are arranged in concentric circles known as orbitals, or energy levels; each of these orbitals are at a specific distance away from the nucleus, and this distance remains the same throughout all specimens of the species of atom. We say that the energy levels are quantized--energy comes and goes in specific packets, or quanta, and this moves the electrons to discrete positions in the atom, or orbitals (energy levels). This forms the basis of the entire field of quantum mechanics, but we don’t need to go into all that. The important thing is that electrons can accept these packets of energy in the form of light (photons), and rise to a higher orbital, where it remains in an excited state. Sidenote: By the theory of wave-particle duality, photons exhibit the qualities of both waves and particles; when describing their behavior as waves, they are more often referred to as electromagnetic radiation, while in particle form, we call them photons. Energy is added by the photon, resulting in the photon disappearing and the electron, having absorbed the photon’s energy, being elevated to the next energy level. From there, it will eventually return back down to a lower orbital, releasing energy, again in the form of a photon; this is because it takes energy to keep electrons aloft in higher levels. Electrons are more stable at lower levels (similar to how we feel safer low on a cliff than on top of one) and will then try to return to a lower level.
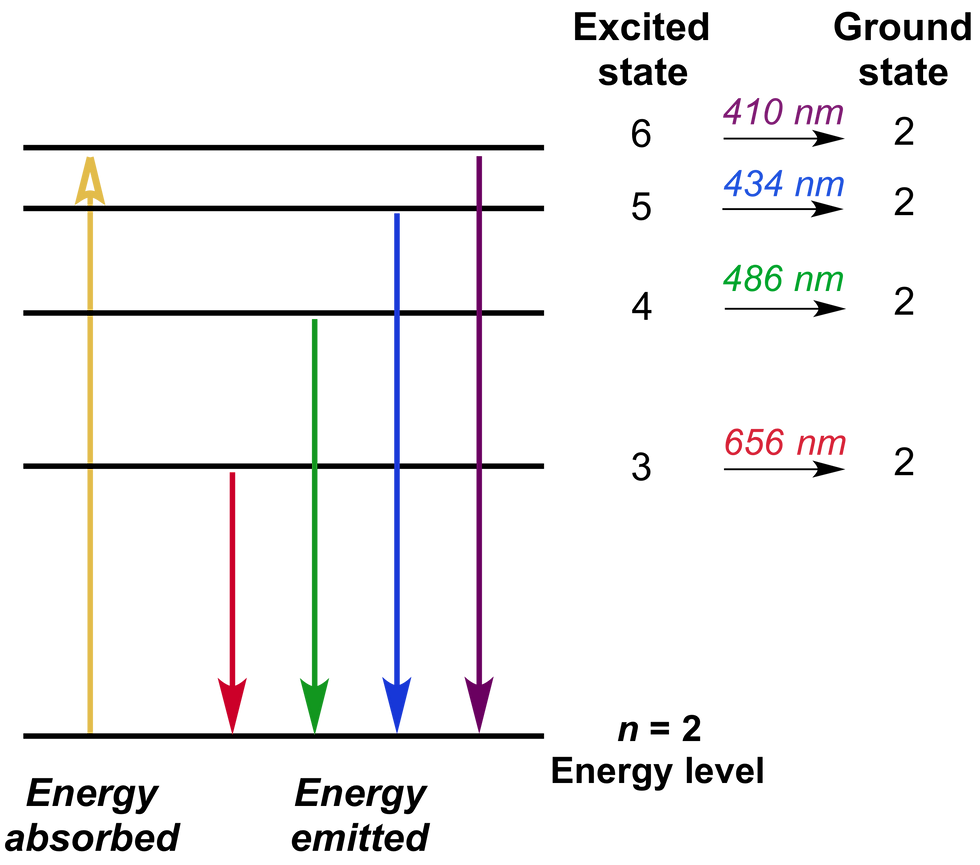
The important thing to note here is that the distance and the energy difference between the energy levels are constant (depending on the atom). Relative to how far the excited electron falls, it will release that much energy in the form of a photon with a frequency corresponding to that energy. The photon released also depends on which energy level the electron falls to; electrons that fall from upper energy levels to the first one will emit ultraviolet radiation and above, while those that fall to the second energy level emit visible radiation, those falling to the third emit IR radiation, and so on. What determines the finer frequencies of these photons are the energy levels it falls from - for example, an electron that falls from the fourth level to the second level will emit a photon of a higher frequency (and energy) than one that falls from the third, because more energy was input putting the electron there in the first place. Generally, in nature, this happens over a period of time in a phenomenon known as spontaneous emission; photons of various frequencies are emitted in random directions over an extended period of time. Fluorescent bulbs depend on this technology as well--energy is inputted as invisible UV light, and the gas in the bulb absorbs this, and in turn releases photons in the visible spectrum.
Lasers take this one step further. Stimulated emission is when a photon of a certain frequency meets an electron at an appropriate quantum level, the electron will fall to a lower level and release a photon with the exact same frequency as the incident photon. This occurs because as the electron is hit by the photon, it enters a transitory state where it acts as an electric dipole; this allows the transitory particle to oscillate at the same frequency as the electromagnetic field. (AKA the photon - remember, by wave-particle duality, a photon is also an electromagnetic wave. These terms will be used interchangeably throughout this article) Thus the new photon has a drastically increased probability to be of the same frequency as the incident photon. This increased chance is, generally, an almost certainty - most lasers emit light in frequencies that are, for all practical purposes, identical.
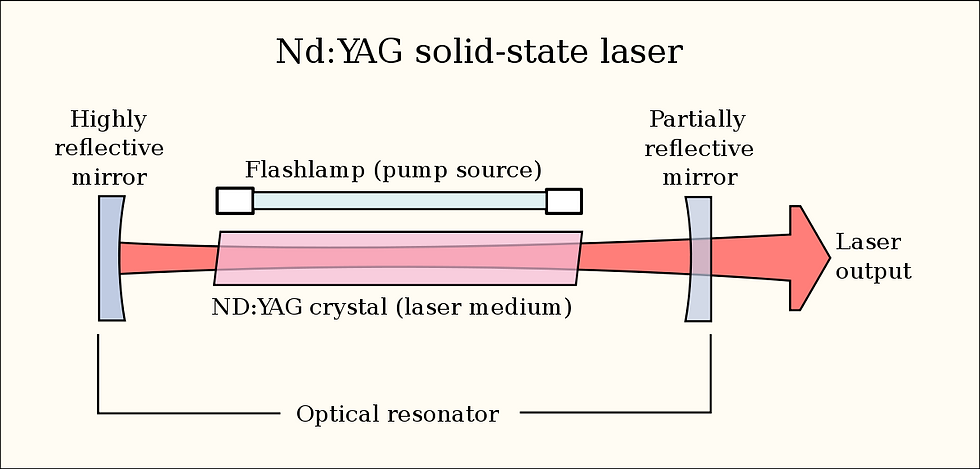
Returning to the laser’s structure, we left off at something called a gain medium. The energy pump will pump energy (photons) of a certain frequency into the gain medium, upon which the photons will, thanks to the mirrors, bounce around within the gain medium and amplify itself, causing massive amounts of stimulated emission. Photons of the same frequency will meet other electrons in the medium and cause more photons of the frequency to be emitted, and this is repeated in the gain medium in a recursive cycle for the duration of the laser’s activation. This part gives the LASER its ‘A’. Light is Amplified by Stimulated Emission, creating Radiation of a specific frequency. The amplified light does not stay in the device, however: one of the two mirrors, again, will be partially transparent; it is through this gap which photons will escape, and create a laser beam.
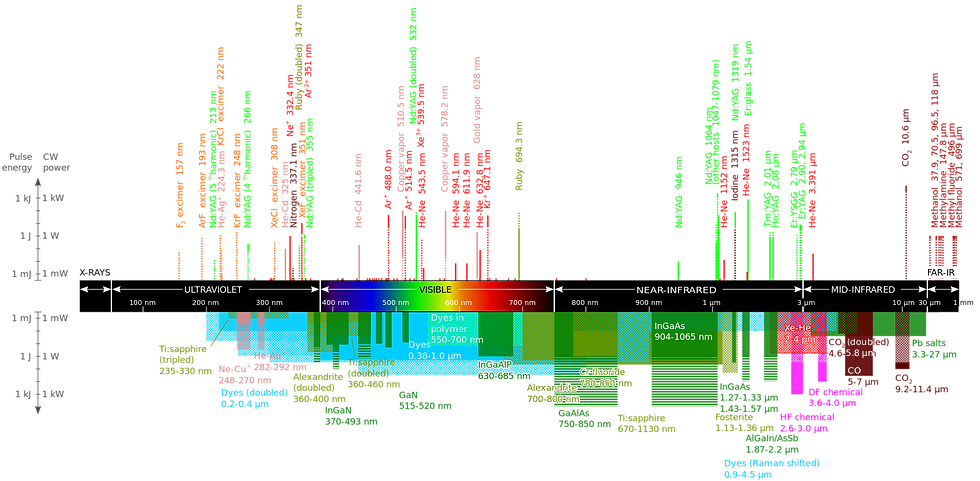
Gain mediums come in a variety of states and forms--solid mediums exist, as well as gas gain mediums, organic dyes, etc. The identity of this medium will determine the frequency of the resulting wave. The diagram outlines various mediums and their respective frequencies.
Laser Weaponry
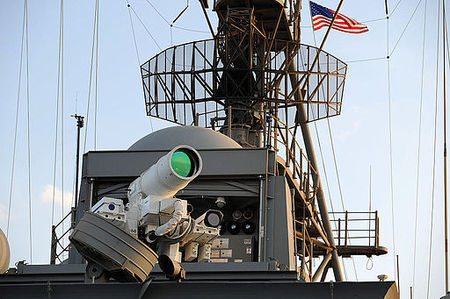
By now, you’re familiar with what a laser is and how it works - so it’s time to get to the fun part.
The United States military, among others, has worked on laser weaponry for some time now, and successful examples exist, such as the LaWS (Laser Weapon System, pictured left), mounted on the USS Ponce, or the Boeing YAL-1, an airborne laser system designed to shoot down ballistic missiles (this has since been discontinued). Unlike laser pointers, laser weapons generally emit only brief, intense pulses. Their destructive effect comes from the shockwaves produced in the target by the rapid deformation caused by the evaporation and expansion of the surface from the heating effects provided by the laser. These are rather difficult to build right now, however - such lasers typically require power on the order of dozens to hundreds of kilowatts(1 kilowatt = 1,000 watts)*** and on anything other than a stationary emplacement (such as tanks, planes, etc.) such power levels are very difficult to accomplish with current technology. For comparison, your average desktop computer runs at a power of about 500 watts. This is just for the lasers we have today, which operate at visible or ultraviolet frequencies--current laser weapon systems operate at the infrared (which transfers heat). For more exotic (and so far, theoretical) devices such as X-ray lasers and gamma lasers, the energy needs grow exponentially. For such devices, power needs to be supplied at the rate of nearly one watt per atom (of the gain medium), which, considering the billions of atoms involved in a tiny fraction of any substance, is unrealistic if not impossible.
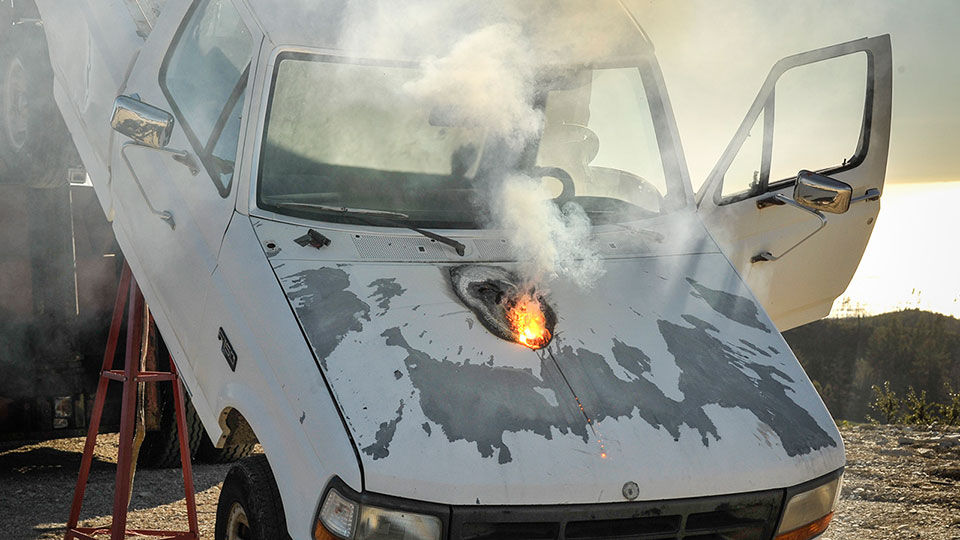
Now, laser weapons in movies are typically depicted as gun-like devices with barrels that shoot projectile-like bolts of light. This is incorrect for a variety of reasons. Because lasers emit photons, the beam of light travels at the speed of, well, light. That’s 299,792,458 meters per second. If the microsecond pulse of the beam is visible at all, it will be there and gone in an infinitesimal fraction of a moment. That being said, laser beams themselves aren’t generally visible; if you’ve seen a laser pointer, you’ll see what I mean. You can only see the dot that it creates. That’s because the photons are directed in only one direction, and for someone to see light photons need to physically enter the eye. Unless the laser is pointed into the eye, that won’t happen (don’t ever do this!). What we do see is the photons scattering off whatever surface the laser is pointed at. If you were to take a bunch of water and spray it in the laser’s path, you would be able to see the beam - or at least, the beam scattering off the water molecules. (This is the only reason laser beams are visible in nightclubs and concerts; smoke pots are activated for effect, and the vapor that fills the area as a result scatters the light, resulting in visible colored beams.) My point being that, without some form of particulate matter to scatter the light, you wouldn’t be able to see the beam itself, much less in a (relatively) slow projectile-like form that flies across space; what you would see is a small point of light (or not, depending on the wavelength) appear on the target for the briefest of moments, followed by a flame, a cloud of metal vapor, and a rapid detonation as the shockwaves from the sudden heat propagate through the target’s surface. Above, you may notice parts of the target truck’s hood is gray - this is where the paint has spalled off from the expansion shockwave.
A laser would neither have nor need a barrel in the conventional sense. Once it leaves the gain medium, it might require a series of lenses to focus it, but long, slender barrels, such as those seen on the tips of a wayward X-wing, would be wholly unnecessary unless the entire barrel is filled with gain medium; this would, however, most likely be cost-prohibitive and needlessly add mass to the setup.
Another consequence of the speed of the laser beam is that it is quite impossible to miss. As long as the weapon is pointed accurately at the target, unless the target itself is traveling near the speed of light, or really, really far away, the light will travel too fast for any kind of evasion to occur. Since modern laser weapons are mounted on swivels, and are autonomous systems, operated by computers that can keep a laser trained on a target to many hundredths of a degree, it is, quite simply, impossible to miss. With such technology available, it seems all but foolish to mount laser weaponry on the tips of wings where it will only fire in the direction the vehicle is pointed - this will most likely massively detract from a laser weapon’s efficacy.
Lastly, laser weapons as we know them today require immense bursts of power, meaning they cannot be mounted on all but the largest of platforms that are capable of supplying the requisite energy. To date, such weapons with significant destructive capability have been mounted on ships and converted passenger craft, vehicles large enough to carry the capacitors necessary to muster dozens of kilowatts of power. Meaning that handheld laser weapons will scarcely be able to have the destructive capacity of its more traditional, projectile-based counterparts (guns), unless the soldier lugs around with himself an enormous battery pack. Lasers, by (modern) design, are quite bulky and require much volume to carry around. Which is why, laser weapons as depicted in movies are quite impossible - compact, handheld or fighter-plane mounted lasers shooting projectile-like bursts of light that miss are quite outside the realm of physical plausibility. Laser weapons in the modern age will be bulky affairs, mounted on large vehicles, which, despite their size and relatively unimpressive visual effect, will nevertheless be deadly accurate and undoubtedly effective systems due to their cost-effectivity and near invisibility when fired.
Conclusions
Laser weapons, should they reach a more effective form, are certainly promising; unlike conventional projectile weapons, they do not need projectiles, which means the metal and energy devoted to producing them may be directed elsewhere. In fact, all it needs is a power source, since its means of delivering energy consists of nothing but light, and thanks to this the cost-per-shot of a laser weapon will be substantially lower than that of a projectile weapon of equivalent destructive power. These are only a few among many of the laser’s wide-ranging benefits and uses; and even now, more and more of the world is dependent on them. While the movie lasers we all know and love are, in fact, a physical impossibility, we can take comfort in the fact that we have something almost as fascinating around us.
It does look a lot cooler in the movies, though.
*Solar radiation is mostly (52%) infrared light (or the primary carrier of heat). The remaining proportion is a mixture of visible light (45% - what we see), and ultraviolet (UV) light (3%). Higher frequencies of UV are filtered off by the ozone layer. Radioactive radiation is, in other terms, gamma radiation (no, it will not turn you into the Hulk, but it will kill you nonetheless), with a wavelength of under 0.02 nanometers.
*The reason why ultraviolet light causes skin cancer, X-rays are dangerous and radiation will kill you is really because of their short wavelengths (or high frequencies). Starting from higher-frequency UV onwards, the waves become energetic enough, and the wavelengths become short enough, that they can affect your cells internally, possibly leading to mutations such as cancer or killing them outright. Thankfully, the ozone layer filters out much of the dangerous UV (this is one of the reasons why the ozone layer is considered so important), X-ray scans are brief, and we bury radioactive waste in deep pits far from ourselves, otherwise we’d all be dead, or have a third eye.
**Disks operate behind a simple principle - disks are physically stamped with a machine, so that on a micro(nano)scopic scale, there are many, many small and large indentations in just as many concentric circles around its center which comprise the data. As the disk spins, the indentations are ‘read’ by a laser diode in the disk drive. Modern Blu-ray drives’ laser diodes emit blue light (405 nm); this is a smaller wavelength than the red light emitted by red laser diodes (wavelength 650 nm) used for DVD drives. Smaller wavelengths mean that smaller indentations can be read; this allows disk manufacturers to store more data on the same surface area, hence the larger capacity of the Blu-ray. DVDs store more data than CDs on the same principle - CDs are read with infrared light (wavelength 780 nm))
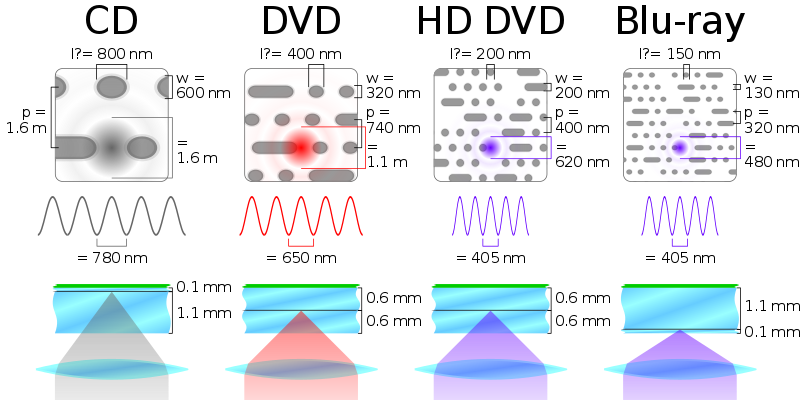
***Power is different from energy - power is a measure of how much energy is expended per second, and has units of Watts (W). (Energy has a unit of Joules, or J.) Energy is not so much a problem with laser weapons as how much energy the laser can put through in a given amount of time - this is why capacitors are crucial to laser weapons, even more so than batteries or a similar power source. (Capacitors are devices that store current and release it rapidly.)
━━━━━━━━
Bibliography:
- Fritzsche, Hellmut, and Melba Phillips. “Electromagnetic Radiation.” Encyclopædia Britannica, Encyclopædia Britannica, Inc., 18 Oct. 2017, www.britannica.com/science/electromagnetic-radiation.
- Hecht, Jeff. “Laser.” Encyclopædia Britannica, Encyclopædia Britannica, Inc., 13 Feb. 2018, www.britannica.com/technology/laser.
- How Lasers Work, lasers.llnl.gov/education/how_lasers_work.
- “Laser Weapon System.” Wikipedia, Wikimedia Foundation, 16 Mar. 2018, en.wikipedia.org/wiki/Laser_Weapon_System.
- “Laser.” Wikipedia, Wikimedia Foundation, 16 Mar. 2018, en.wikipedia.org/wiki/Laser.
- Libretexts. “Electromagnetic Radiation.” Chemistry LibreTexts, Libretexts, 21 July 2016, echem.libretexts.org/Core/Physical_and_Theoretical_Chemistry/Spectroscopy/Fundamentals_of_Spectroscopy/Electromagnetic_Radiation.
- “Stimulated Emission.” Wikipedia, Wikimedia Foundation, 11 Mar. 2018, en.wikipedia.org/wiki/Stimulated_emission.
Pictures:
- https://lumiere-a.akamaihd.net/v1/images/resistance-x-wing_9433981f.jpeg?region=0%2C5%2C1560%2C781
━━━━━━━━
Comments