Written by Harry J. (GAFL'19)
Edited by Jenny C. (VHS'19)
āā February 26, 2018 āā

Overview
The Red Planet has long been a source of intrigue and scientific curiosity, and given its proximity to our own planet - and its potential habitability - it is no big wonder that this is the case. No individual in history, however, has come as close to satisfying that curiosity than Elon Musk.
For those unfamiliar with this individual, Elon Musk is the founder and CEO of, among other enterprises, SpaceX, which has revolutionized the space travel with fully reusable rocket systems, cutting costs to space travel drastically and demonstrating that the barrier to entering the space business is not so high after all. Muskās aspirations have since grown with his expertise, however, and in the early 2010s, he announced that he will put humans on Mars by no later than 2024, with the ultimate goal of establishing a colony of millions of people before the end of the century. Naturally, such ambitious plans have captured the imagination - and inspired the skepticism - of many. Through this exploration, we will delve into the reasons behind both, and determine for ourselves which of the two sentiments is justified.
āāāāāāāā
The Mission
The Red Planet
To answer this question, let us first explore the planet in question. Mars is the fourth planet from the Sun, preceded by Mercury, Venus, and Earth, and followed by Jupiter, Saturn, Uranus, and Neptune. It is the second-smallest planet in our neighborhood, yet it is home to some of the most stunning geographical features around, such as Olympus Mons, our solar systemās tallest mountain, and Valles Marineris, the solar systemās longest and deepest canyon. Impact craters from meteors that the thin atmosphere - 0.6% by density - could not burn up pockmark the surface. There are red skies and blue sunsets. What water there was, the thin atmospheric pressure has sublimated or frozen, although massive subterranean reserves are known to exist. Moreover, Mars has long since lost its magnetosphere, which leaves the planet and its atmosphere defenseless against radiation and solar winds. It has a surface gravity just above a third of Earthās (0.38 gās, to be exact), and its surface is abundantly covered in iron oxide, resulting in its signature red color that only infrequently greets our own eyes, accustomed themselves to the lush greens and blues (or grays, depending) of Earth. Evidently, Mars hosts an environment much different from Earth - and yet, in many respects, the planet could have been Earthās little brother.
Its surface is layered with canyons, mountains, and riverbeds, features that suggest Mars may have once hosted rivers and lakes on its surface. It has a thin atmosphere (0.6% of Earthās by density) composed primarily of carbon dioxide (96%), which once might have been thick enough to sustain an ecosystem like Earthās, but has since boiled off into space and into the ground. Marsās soil is rich in minerals, to the extent that it may foster life should other conditions (temperature, the presence of organic matter, pressure, etcā¦) allow. The planet is orbitally inclined - it spins, with twenty-five hours in a day, on a tilted axis, which means to a limited extent, it has seasons of its own. Had things gone differently many millions of years ago, Mars would have become a terrestrial planet much like our own.
Despite its desolate, seemingly alien landscape, within our solar system, it provides the most attractive prospects for habitation due to these similarities. In light of this, numerous plans and suggestions for reaching and staying on the Red Planet have been proposed, but none are quite as comprehensive, ambitious and close to fruition as that of Elon Muskās.
Delivery System
Muskās plan hinges on a delivery system dubbed the Interplanetary Transport System, or the ITS for short. This is comprised of three different subsystems: the launch vehicle, which is essentially a scaled-up version of the reusable Falcon 9 rocket, the passenger spaceship itself (known somewhat confusingly as (also) the ITS, or the Interplanetary Transport Spaceship; the passenger spaceship will be referred to as the ITS for brevity), and the refueling tanker.
The project is based on a 9-meter diameter rocket; it is somewhat smaller than the venerable Saturn V, which had a diameter of 10. At the core of the ITS rocket is the booster, the column of carbon fiber, methane, and oxygen that will push the spacecraft into orbit, and return to the surface to subsequently launch the Tanker (explained shortly).
In an approach virtually identical to its predecessor, the Falcon 9 (a commercial rocket in operation, designed by SpaceX - currently the only rocket with a fully reusable booster) relying on its own engines instead of parachutes for reentry as has been traditionally done for anything re-entering the atmosphere prior to SpaceX. In this sense, the booster will operate almost identically to the Falcon booster already in use today. Notable is its use of grid fins - these are stowed during ascent, where their low profile will provide minimal drag, and unfolded outwards during descent, where they both provide some measure of air resistance to slow the booster as well as providing a larger net control surface as compared to traditional fins. Considering these aspects, along with the technical expertise the company has demonstrated in the Falcon 9ās systems, the booster is a conceptually sound design. Pictured below is an artistās conception of the booster returning to Earth after lofting its payload.

The ITS is to utilize a completely new engine for all of its operations. Currently, the Falcon 9 rocket uses 9 SpaceX Merlin engines, which burn a mixture of RP-1 (rocket-grade kerosene), and liquid oxygen for its thrust. The Raptor engine - as named by its engineers at SpaceX - will instead rely on liquefied methane to replace RP-1, for reasons explained later. Typically the longest, most crucial and most expensive part of designing a new delivery system, the engine is the backbone of the rocket, and by extension, reaching Mars as a whole - as such, SpaceX has gone to great lengths to make the Raptor engine one of the most competitive to date with unparalleled thrust and efficiency.
The booster is to carry 42 of these Raptor engines, which would offer a monstrous 52.7 meganewtons (52,700,000 newtons) of thrust (for comparison, the Saturn V booster only managed 36 MN, an impressive number in itself - for perspective, the gravitational force exerted on a blue whale is 1.4 MN), and thus promises to be one of the most powerful first-stage boosters in history.
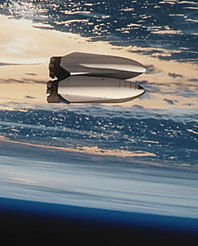
The ITS Tanker is an unconventional, but necessary, addition to the list. Traditionally, fuel loads for whatever mission was at hand were carried along with the payload, meaning probes bound for distant destinations were designed with special care to how much fuel it could carry on the ascent. The Tanker vehicle changes this completely - it allows the spaceship itself to expend fuel during its ascent, meaning a) a lighter load for the engines as more fuel is used and b) no need for engineers to worry overly about fuel economy on the way up, as fuel is guaranteed by refueling as needed. The Tanker vehicle itself is a large, unmanned tank of methalox. This is necessary because once the actual spaceship is pushed into orbit, it will have expended most of its own fuel in the process - the Tanker is then sent back up with the recovered booster (which, in mere minutes, will be back on the surface after launching the ITS), and the fuel (up to 380 tons) in the tanker will be transferred to the spaceship up to five times (for a total of about 1900 tons), which then has sufficient fuel to continue to Mars. To the left is an artistās impression of the refueling in progress above Earth.
The Interplanetary Transport Spaceship (also āITSā) forms the crux of the ITS infrastructure. The spaceship is at once a second-stage booster, an interplanetary spacecraft, and a colonial base. Both the spaceship and the tanker will also feature SpaceXās proprietary Raptor engines, albeit with different expansion ratios*, an uncommon arrangement in spacecraft (where so far, the upper and lower stages of preceding rockets have used different engines). These engines will facilitate the trip to, and landing on, the surface of Mars, where following a horizontal descent and aerobraking maneuver the ITS will land vertically much like the first stage booster. Once it is landed, it will turn into a base for the colonists, who will then oversee operations to establish a permanent presence on the Red Planet. This must be done judiciously and efficiently, as the respective synodic periods** of Mars and Earth allow for only nine opportunities for transit from 2020 to 2037. Should a return mission ever become necessary, the ITS is expected to take off from Mars on its own (only a few bodies in the solar system other than Earth are massive enough for rockets to require a dedicated first-stage booster to take off (massive bodies exert more gravitational force on objects, which means itās much harder to take off from massive planets)).
Mission Profile
A typical mission to Mars, based on Muskās planned infrastructure, will look something like this: from Pad 39A at Cape Canaveral, Florida (where, incidentally, the Saturn V missions were launched from as well), the ITS booster lifts off with the spaceship carrying close to a hundred colonists. Once the booster separates, it will cut its own orbit short and re-enter the atmosphere, landing back at Cape Canaveral and leaving the spaceship in orbit. (SpaceX has already proven its capability to land spent boosters with great accuracy on floating barges; landing them back on the pads they launched from is only a small step further.) Before the ITS actually heads on its way to Mars, its tanker and booster will go through a series of up to five launches, landings, and relaunches. The purpose of this process is to ensure that fuel is completely transferred from the tanker to the booster.
Once this is complete, it is time for the ITS to head to Mars. After a brief burn in Earth orbit, the spaceship will gain enough velocity to escape Earthās gravity well, and propel itself towards Mars. Contrary to popular belief, engines are not fired the whole way to a spacecraftās destination - in Earthās atmosphere, it is necessary because air resistance will slow down the vehicle. But in space, where no such obstacle exists, in many cases, only an initial boost from the engines occur, and the spacecraft coasts at a fixed velocity the rest of the way. (This is not the case for Hall effect thrusters and a few other engine types, but more on this in another article.) It will take 80~150 days to reach the orbit of Mars, depending on which synodic period the spacecraft is traveling in. The resultant orbit at Mars will skim the top of the planetās atmosphere. As the spacecraft approaches the planet and comes into contact with the atmosphere, air resistance will slow down the ITS so that the orbit sinks until it intersects the surface of the planet. At this point, the spaceship will be oriented perpendicular to its direction of motion so that drag - and deceleration - will be maximized. The spaceship will be traveling at speeds nearly 8.5 km/s at entry, and the rapid deceleration means its passengers will experience up to 5 gās of force. To the left is an artistās conception of Mars atmospheric entry.
Once a sufficient velocity has been reached, the spacecraft (aircraft, at this point) will turn fully retrograde (backward) and fire its central column of 3 engines, upon which it will slow down and land vertically in a fashion akin to the first-stage booster. Once it lands, the next phase of the mission, in-situ resource utilization, kicks into effect.

In-Situ Resource Utilization
An interesting feature of the ITS is that it is a departure from previous trends in rocketry, relying on methalox*** (a moniker for liquid methane + oxygen fuel mixture) instead of the traditional liquid hydrogen or RP-1 fuels. This is primarily because of the resourceās reusability and ready availability in the Martian environment. Along with untold kilotons of frozen water and buried minerals, methane (CH4) is abundant on Mars in the of methane clathrate, a crystal in which methane molecules are trapped in an ice-like lattice of water; the methane molecules may be liberated from the lattice, separating the clathrate into its constituent parts, methane (fuel) and water (for drinking, farming, cleaning, etc.). Other pathways include synthesizing the carbon dioxide in the atmosphere with hydrogen, a process (made possible by something known as the Sabatier reaction****) that will result, again, in methane and water, both useful products for prospective colonists. Another plus is that the reaction is exothermic (-165 kJ/mol), and can thus generate heat, and by extension, energy, which may be used to power other components of the colony, be it laboratory equipment or temperature. Through either of these methods, once obtained on-site after landing, the raw fuel can then be further processed and liquefied into a usable form, which will allow the ITS to return to Earth.This concept of using native resources for fuel is known as In-Situ (Latin, on-site) Resource Utilization, or ISRU for short. This process can not be accomplished with other fuels such as hydrogen or kerosene, as neither chemical exists in feasibly obtainable levels on Marsā surface, at least that we know of. Either way, Marsā own natural resources adequately would provide fuel for round-trips if one were to establish a propellant plant on the planet, which, naturally is a pivotal part of Elon Muskās plan.
Water, again, is abundantly present, albeit in frozen form, both as part of crystals bound to other compounds or as pure ice. Once this is liquefied, water will be in abundant supply for drinking or farming purposes, provided that the landing takes place close to a viable source. Because Martian soil contains adequate levels of minerals like magnesium and calcium, if SpaceX decides to transport seeds and bacteria in the ITS, Martian colonists could conceivably establish hydroponics farms to sustain themselves, a crucial step if we are to eventually establish a permanent colony. Should the transport system reach completion, there is no reason transport to and settlement on Mars cannot be accomplished, given the technology we have today. (For the extra curious, Iād recommend The Martian by Andy Weir; while a fictional work by nature, it is, for the most part, an accurate and realistic representation of what a base on Mars would resemble, based on current technology. Also, itās a really good book.)
Conclusions
Based on the information laid down about the mission at hand, Elon Muskās personal resolve, and the technology and experience backing it, Muskās plan to go to Mars and the ITS infrastructure he has proposed to enable it seems sound in all aspects. And, to that end, funding is the only real obstacle the enterprise faces, considering that, despite the solid foundation of the plan, extraterrestrial exploration is a highly speculative business. There is also the human aspect in preparing the mission, as such an undertaking will likely take years and require a substantial commitment from the colonists, considering the psychological and physical stress of being the first specimens of humanity on a planet millions of kilometers from their own, separated from home by vast swathes of space and time (transit to and from Mars takes months at its shortest). But individuals who can weather such conditions undoubtedly exist - considering the pool of seven billion we have to choose from - and should Musk be successful in securing the monetary resources to support this project, the only thing that can cause its downfall is sheer astronomical bad luck. Unfortunately, our generation of humanity was born somewhat too late to explore the world, and born much too early to explore the universe; but thanks to Elon Musk, we may be born just in time, perhaps, to explore Mars.
āāāāāāāā
*Expansion Ratios: Rocket engines are composed primarily of fuel tanks, where the fuel is stored, combustion chambers, where the fuel is burned, the pumps and pipes that transport the fuel there, and the nozzle through which the combusted fuel is ejected as reaction mass to propel the rocket forward (Newtonās third law). Expansion ratios are an intrinsic quality of the rocketās nozzle, and it deals with the ratio between the area of the nozzleās neck (the narrowest part) and the exit plane, or how wide of a āflareā the nozzle has. This is important because the exhaust jet is almost always supersonic - this means the pressure of the exiting gas may be wildly different from the ambient pressure. As a consequence, as altitude changes a nozzle may be under expanded (jet pressure exceeds ambient - top), ambient (appropriately expanded - second), or overexpanded (jet pressure is far lower than ambient - bottom two). Deviations in either direction may result in unstable exhaust jets and loss of efficiency, which is why many rockets with multiple stages use different engines with distinct expansion ratios designed specifically for the altitudes they will operate in. SpaceX differs in that it keeps the same engine with different nozzles and expansion ratios, with an expansion ratio of 40 for the first stage (which will operate in the lower atmosphere), and 200 for the passenger spacecraft (which will operate in thin or nonexistent ambient pressures).
**Synodic Periods: In terms of mean distance from the Sun, Earth and Mars are separated by 80 million kilometers of space (Earthās distance from the Sun is 150 million kilometers on average, and Marsā distance from the sun is 230 million kilometers on average). This means their orbital periods will differ, with Mars taking far longer to orbit the Sun than Earth (687 days, compared to Earthās 365). However, this means that, much like the hour and minute hands of a clock that comes together every hour, there will exist a fixed interval at which the two planets will line up with the sun and reach a position in which they are closest to each other, shortening the time necessary for transit. This interval is known as a synodic period, and represent ideal opportunities for transit to other bodies in that the relatively short distances will allow for minimal consumption of fuel and time. The synodic period between Earth and Mars is about 2.135 years, which means every two years or so the two planets will be spaced closer together in ideal position for transit.
***Methalox: Rockets differ from atmospheric jet engines in that the oxygen used to combust the fuel does not originate from the atmosphere but from internal reserves of liquid oxygen (combustion, by definition, requires oxygen to occur), which means ārocket fuelā is actually comprised of two components, the fuel, and the oxidizer. Oxidizer has traditionally been liquid oxygen or other oxygen-rich compounds such as nitrogen tetroxide or hydrogen peroxide. The fuel component has been more varied, with compounds such as RP-1 (refined kerosene), liquid hydrogen, or hydrazine (N2H4, used in conjunction with nitrogen tetroxide) being the most common choices. Methane is a relatively new addition to the family, and in the perspective of a Mars mission, it has advantages in that it is easily synthesized on Marsā surface, and is far denser and less volume-demanding as liquid hydrogen, the propellant of choice for many modern rockets. Such engines, with two separate chemicals (fuel and oxidizer) that are combusted together, are known as bipropellant rockets, in contrast with monopropellant rockets*** and the much rarer (and more complex) tripropellant rockets.
***Monopropellant rockets use only one chemical in their propulsion, and thus rely on chemical energy within the molecule, and not in the interactions between them. These are generally not used for primary engines, instead utilized in attitude thrusters in the vacuum where monopropellant thrusters are arrayed at various points around the spacecraft to change its orientation.
****Sabatier Reaction: CO2 + 4H2 ā CH4 + 2H2O. The process discovered by Paul Sabatier, carbon dioxide, and water reacted at temperatures of 300~400 degrees Celsius in presence of nickel catalyst. The reaction is exothermic, with an enthalpy of -165kJ/mol.
āāāāāāāā
Bibliography:
- āInterplanetary Transport System Booster ā SpaceX | Spaceflight101.ā SpaceX Spaceflight101, spaceflight101.com/spx/its-booster/.
- Spacexcmsadmin. āMars.ā SpaceX, SpaceX, 20 Sept. 2016, www.spacex.com/mars.
- āSpaceX | Spaceflight101 ā Spaceflight101.ā SpaceX Spaceflight101, spaceflight101.com/spx/.
- Writer, Mike Wall Space.com Senior. āSpaceX's Mars Colony Plan: By the Numbers.ā Space.com, www.space.com/34234-spacex-mars-colony-plan-by-the-numbers.html.
Pictures:
āāāāāāāā
Comments