Written by Yuju P. (GAFL'18)
Edited by Jaemin Y. (KIS'19)
━━ April 8th, 2018 ━━

The Juno Mission
Nasa’s Juno spacecraft arrived at Jupiter on July 4th, 2016, celebrating American Independence Day. It has been orbiting Jupiter in polar orbit ever since, providing scientific data and beautiful close-up images of Jupiter. Juno is the first spacecraft to go out into the outer solar system on solar power. The public engagement is one of the highest; anyone with an email account can suggest and vote on https://www.missionjuno.swri.edu/junocam on which part of Jupiter should Juno’s JunoCam should take a picture of, which is actually being followed by the Juno mission team. Why is there so much excitement over this planetary mission to Jupiter, which has already been explored in the past by Galileo? How will the Juno mission benefit the humanity?
Before trying to answer these deep questions, here is a basic overview of Juno spacecraft, according to NASA.

‣ Gravity Science: Maps Jupiter’s gravitational field. The non-uniformity of Jupiter's density causes slight changes in the velocity of the spacecraft, which will be detected and used to backtrack the gravitational field.
‣ Magnetometers: Map Jupiter’s magnetic field. Attached at the far end of the spacecraft to minimize the interference from its own electronic devices.
‣ Microwave Radiometer: Measures the composition of Jupiter’s inner structure, including water and ammonia.
‣ JEDI, JADE, and WAVES: Study Jupiter’s auroras and magnetosphere, a region around a planet where the magnetic field is significantly strong enough to affect charged particles’ paths. Jupiter’s magnetosphere is large to the extent that if it was visible to human eyes, it would be similar to the size of our moon while it is about 1636 times far away from Earth than the moon.
‣ UVS and JIRAM: Take infrared and spectra images of Jovian aurora and atmosphere in the range of 70 to 205 nm (Blau).
‣ JunoCam: The first to take polar images of Jupiter. The public can engage in deciding JunoCam’s target points.
The functions of all these instruments may seem independent of each other, but what they are doing is essentially the same: to study the chemistry and physics of the gas giant’s atmosphere and deep structure (No biology included, unfortunately, as harsh conditions on Jupiter most likely make living organisms inhabitable). One of the scientific goals of the mission is to clarify the existence and the features of Jupiter’s core.
Does Jupiter Have a Core?
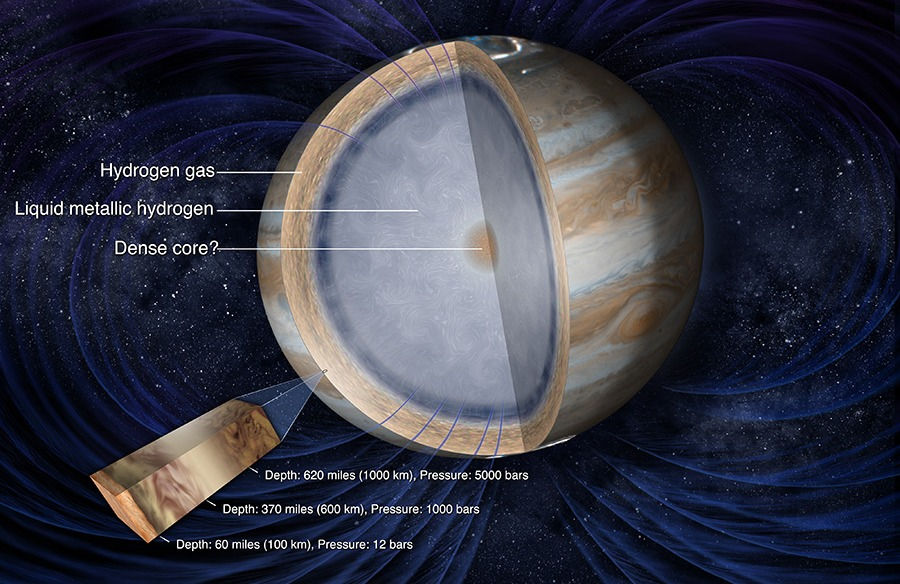
The core of Jupiter, especially its existence, might come as nonsense to some people. Not only do a majority of people believe that Jupiter has a rocky core, but many are commonly taught that way. It is due to the fact that the current standard planet or solar system formation model predicts it to be rocky. However, what is true is that nothing about Jupiter’s core has been proven–only theories or models exist, and even some of the major theories and models deviate drastically in regard to the gaseous planet’s core. Hence, studying one of the gas giants Jupiter’s core will provide scientists more evidence to evaluate and develop the planet’s formation theories, allowing room for a deeper understanding of the origin of our solar system.
The two major models are Core Accretion and Disk Instability. The core accretion is the current standard model for the solar system and planet formation, which predicts gas giants to have rocky cores. On the other hand, the disk instability is not as widely accepted and suggests that gas giants have no core. Each has advantages and disadvantages when it comes to explaining the evolution of our solar system and other stellar systems.
The Core Accretion Model
According to the core accretion model, Jupiter was formed the following way. When clumps of dust and gas collapse under gravitational force, small bodies (called planetesimals) made of mainly dust and gas form within it. These celestial bodies eventually grow over time through aggregations and collisions until they are massive enough and thus their gravitational pull is sufficiently strong enough to form gas layers around its core. There exists a critical mass, which is a point where once it has been reached, the accretion of gas accelerates and only then the formation process happens rapidly.
The core accretion is supported by many pieces of evidence from our solar system. It explains that heavy mass of giant planets would have allowed light gases to be attracted and hence such enormous atmosphere to form. In addition, the expected lifetime of solar nebula is consistent with the core accretion model. The existence of terrestrial planets is also obvious when this model is taken.
Despite the fact that the core accretion is the complete model of all, it does have some weaknesses. The time estimated for Jupiter’s core to have formed ranges from fifty to five hundred million years. However, by then, gas, being light-massed, would have escaped from the system and there would not have been enough gas left for Jupiter to form the atmosphere it has nowadays. Not only that, the formation of Uranus and Neptune would have been unlikely to happen due to the effect of Jupiter’s enormous mass.
The Disk Instability Model
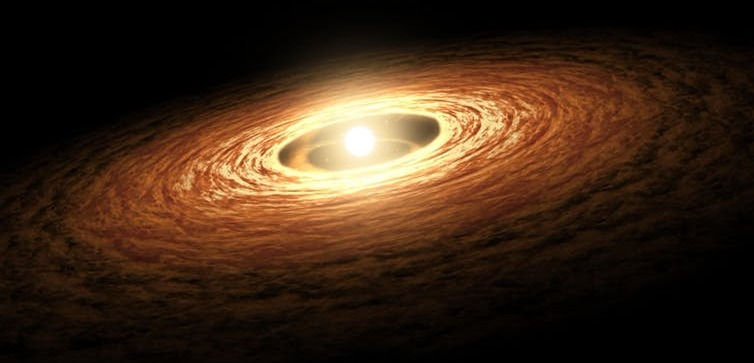
Although less complete, the disk instability model provides alternative solutions to the problems involved with the evolution of gas giants. A rotating disk of dust and gas exists in the vicinity of a young star. Within the disk, rapid cooling causes some particles to condense, which grow and gradually become gaseous planets like Jupiter.
Jupiter formation via disk instability is estimated to take about a thousand years, suggesting far less time required than the core accretion does, even for large planets to form. The disk instability better suits the observational data of Jupiter’s composition as light elements would have been abundant at that time.
One major problem with this model is that the scientific community is not in agreement on the feasibility of such rapid cooling. Although the evolution of gas giants may better be explained, that of terrestrial planets cannot be. Also, the disk must be of high density for planets to form, which raises numerous problems. The disks of such high density are relatively less likely to occur than those satisfying the conditions of the core accretion model. Brown dwarfs can readily form in high densities, while none exists in our solar system. Furthermore, planet migration towards the central star or stars may be very fast, possibly resulting in the destruction of planets during formation. Quantitatively, only around ten percent of various exoplanets studied were consistent with the disk instability model, while the remaining ninety percent were in support of the core accretion model.
Juno’s Impact
Some of the problems involved with both models may be avoided with other processes not mentioned in this article, such as planetary migration or core erosion, but even then the complete picture of the evolution of the solar system cannot be obtained. The fusion of the core accretion and the disk instability model could be the answer, or something entirely unanticipated may be the right picture. Whatever the answer may be, the difficulty of theoretically solving the evolution of Jupiter and the solar system is clear. Data is crucial, and that is what Juno is for. Each theory or model has different predictions on the chemistry and physics of Jupiter. Science instruments onboard Juno are currently revealing Jupiter’s deep secrets that would hopefully guide the scientists to the truth.
Before humans, there was Earth. It may be just Jupiter that Juno is studying, but ultimately it is on the quest to understand the history of the entire solar system. Earth being a part of that system, the current understanding of the history of Earth may improve drastically from Juno’s new discoveries, providing a better insight into the formation of the solar system we are living in.
━━━━━━━━
Bibliography:
- Blau, Patrick. “Instrument Overview.” Juno, spaceflight101, 10 Mar. 2018, spaceflight101.com/juno/instrument-overview/.
- “Mission Juno.” Mission Juno, NASA, www.missionjuno.swri.edu/.
- Greicius, Tony. “Juno.” NASA, NASA, 13 Feb. 2015, www.nasa.gov/mission_pages/juno/main/index.html.
- “Posts about core accretion on Planet Hunters.” Planet Hunters, WordPress.com, blog.planethunters.org/tag/core-accretion/.
- Murphy, Rick Leon. “Dynamics of Extrasolar Planetary Systems .” Astronomy Online - Exoplanet Dynamics, astronomyonline.org/Exoplanets/ExoplanetDynamics.asp.
- Rice, W. and Armitage, P. (2003). On the formation time scale and core masses of gas giant planets. [ebook] ApJL. Available at: https://arxiv.org/pdf/astro-ph/0310191.pdf.
- Matsuo, T., et al. “Planetary Formation Scenarios Revisited: Core‐Accretion versus Disk Instability.” The Astrophysical Journal, vol. 662, no. 2, 2007, pp. 1282–1292., doi:10.1086/517964.
Pictures:
━━━━━━━━
Comments